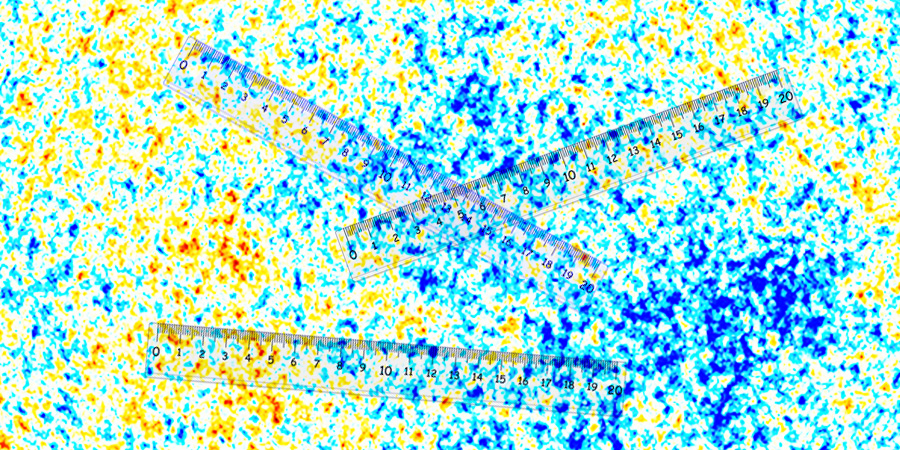
Neutrinos could be the key to understanding dark matter
28-12-2023
The team of researchers from France, Poland, and the United Kingdom has proposed a new hypothesis suggesting that interactions between dark matter and neutrinos could shed light on certain anomalies in the distribution of the cosmic microwave background radiation. The details of the analysis have been described in articles published in the prestigious scientific journals, Monthly Notices of the Royal Astronomical Society: Letters and Physics of the Dark Universe.
Among the known elementary particles, the elusive dark matter particle, sought after for decades, bears the closest resemblance to neutrinos in terms of interaction strength. In the early years of theoretical exploration into the nature of dark matter, there was even speculation that neutrinos produced in the early universe could be responsible for the astronomical phenomena attributed to dark matter. However, they move too swiftly to account for the observed evolution of large-scale structures in the cosmos. Consequently, the prototypical description of dark matter particles shifted towards neutrino-like entities, albeit with a greater mass.
In the meantime, our understanding of neutrinos themselves has grown. We have discovered extraordinary phenomena called neutrino oscillations, which have convinced us that these particles may serve as the most promising gateway to uncover the realms of "new physics". What if, beyond gravity, dark matter primarily communicates with us through neutrinos? The experimental verification of this hypothesis appears to be an almost insurmountable task. Neutrinos, akin to dark matter, can traverse the entire Earth without leaving the slightest trace of their presence. To observe their rare interactions with the matter surrounding us, we require colossal detectors. Yet, how can we observe the impact of neutrino interactions with the equally elusive dark matter? This very conundrum has engaged the minds of researchers within the aforementioned international research team.
A remarkable laboratory for such investigations emerges from studying the early universe via cosmic microwave background radiation. This light was emitted during the formation of the first atoms and currently possesses the characteristics of radiation from an extremely low-temperature perfect black body, approximately 2.7 Kelvin. The minute irregularities observed in the distribution of this temperature across the celestial sphere inform us about the distribution of matter in the fledgling universe. Scientists posit that this distribution was heavily influenced by the presence of dark matter, and the additional interactions between dark matter and neutrinos could significantly disrupt this process. This plausibility is quite strong, given that neutrinos were abundant in the early universe, remnants of the first moments following the Big Bang.
This theoretical observation was initially made over two decades ago, but only recent advancements in the capabilities of observing cosmic microwave background radiation have allowed for attempts at observational verification of this theory. While our understanding of the early universe cannot precisely predict the appearance of the background radiation map, we can astutely describe the anticipated statistical correlations between its properties in different regions of the sky. Presently, we can investigate such correlations on much smaller angular scales, i.e., for minute areas of the sky. In their latest work, the team of researchers demonstrates how this significantly enhances the prospects of discovering the effects of dark matter-neutrino interactions. The potential impact of such interactions appears to remarkably increase with increasingly precise measurements of cosmic microwave background radiation.
Interestingly, observations of cosmic microwave background radiation gathered on larger angular scales do not completely align with those made on smaller scales. This discrepancy has been a source of controversy among researchers for some time. The latest research reveals that this can be explained by introducing non-zero interactions between dark matter and neutrinos. "The presence of such interactions is favored from a data perspective," comments co-author Dr. Sebastian Trojanowski from the international research agencies AstroCeNT, CAMK PAN, and the Theoretical Physics Department at NCBJ. "The statistical significance of this observation is currently below two standard deviations, so the result is not conclusive. It might simply be a chance fluctuation. However, our result aligns with an earlier independent analysis by another group regarding a series of absorption lines in quasar and distant galaxy spectra, known as the Lyman-alpha forest. They too suggested a possible impact of dark matter-neutrino interactions exhibiting the same strength as we perceive. Even if it has nothing to do with dark matter, it is possible that we are on the trail of some genuine phenomenon awaiting explanation."
Further insights into these inquiries will be illuminated by additional observational data collected, for instance, by the Atacama Cosmology Telescope located in Chile, large-scale sky surveys conducted using the Dark Energy Spectroscopic Instrument (DESI), and by the next generation of telescopes studying cosmic microwave background radiation (CMB-S4). Nature unveils pathways for us to explore even the most elusive characteristics of its elementary constituents.
Dr. Trojanowski's research at NCBJ is co-financed by the SONATA BIS research grant entitled "Investigation of Light Particles in the Dark Sector of the Universe," funded by the National Science Center.
The complete research findings are available in the following articles: P. Brax, C.v.d. Bruck, E. Di Valentino, W. Giare, S. Trojanowski, "New Insights on Neutrino Dark Matter Interactions from Small-Scale CMB Observations", MNRAS: Letters, slad157 doi: https://doi.org/10.1093/mnrasl/slad157
P. Brax, C.v.d. Bruck, E. Di Valentino, W. Giare, S. Trojanowski, "Extended Analysis of Neutrino-Dark Matter Interactions with Small-Scale CMB Experiments", Phys.Dark Univ. 42 (2023) 101321, doi: https://www.sciencedirect.com/science/article/pii/S2212686423001553?via%…
Additional information:
Dark matter is a hypothetical form of matter that does not emit, absorb, or reflect light. Its existence is inferred from astronomical observations, which indicate that there is much more matter in the universe than can be accounted for by visible matter alone. Dark matter is necessary to explain many astronomical observations, such as the rotation of galaxies (galaxies rotate at such speeds that, without dark matter, stars at their outskirts should fly apart), the formation of galaxies (galaxies form through collisions and mergers of smaller matter; dark matter can aid these processes by increasing the gravitational attraction between objects), and gravitational lensing (dark masses of galaxies and galaxy clusters can bend light from distant objects, creating a lensing effect). Dark matter constitutes about 84% of all matter in the universe. Its composition and properties are still unknown, but scientists are working to discover them.
Neutrinos are elementary particles with zero electric charge and very small mass. They are so light that they pass through matter without leaving a trace. They are produced in various physical processes, such as radioactive decay, nuclear reactions, and particle collisions. They are present in vast quantities throughout the universe. Neutrinos are important for understanding many physical phenomena, such as stellar evolution, galaxy formation, and the structure of the universe. Despite the extremely low probability of neutrino interactions with other components of matter, scientists rely on directly detecting them.