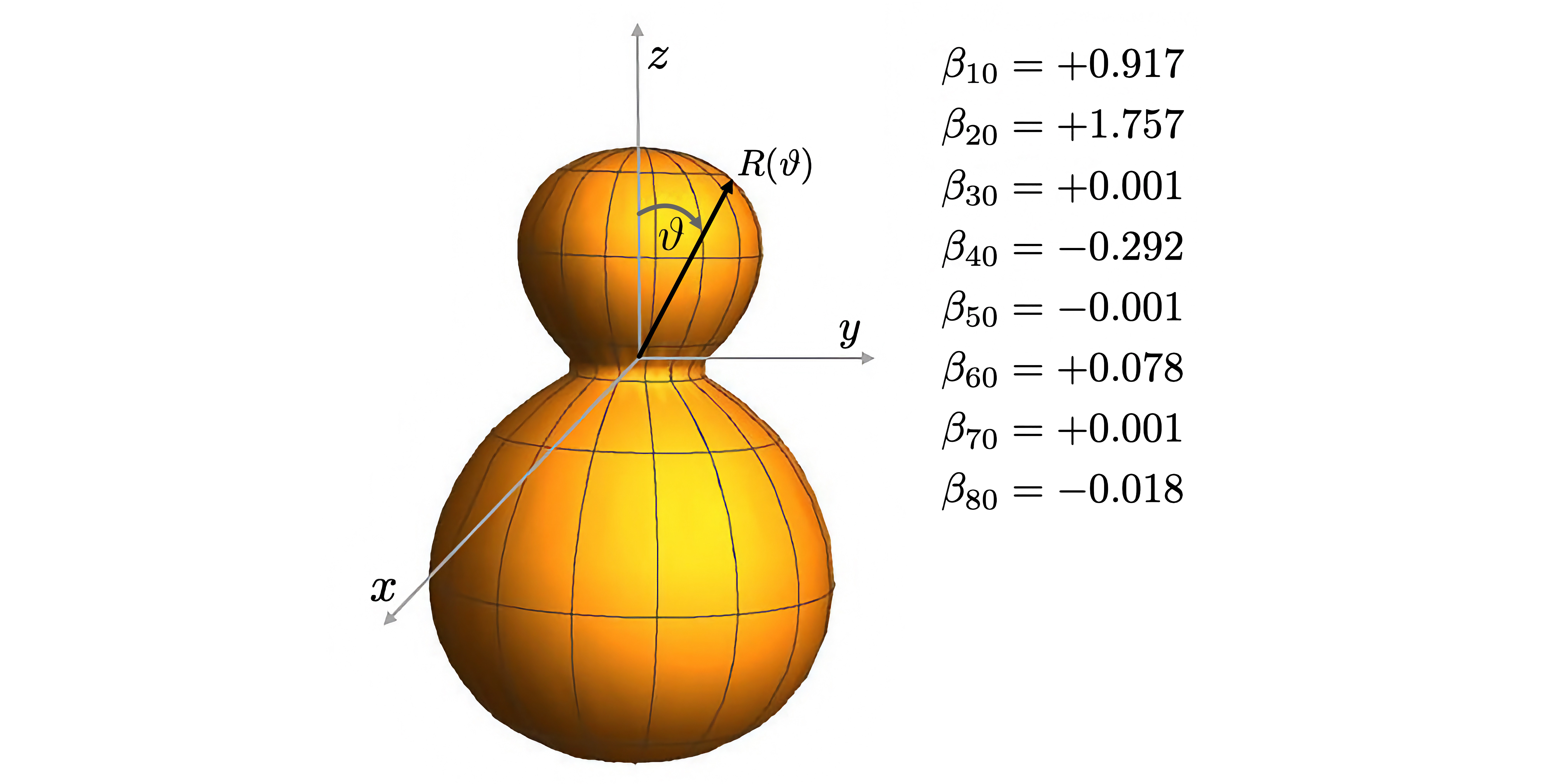
A New Description of Superheavy Nuclei Synthesis
03-07-2024
The heaviest known elements can only be produced under laboratory conditions. For years, nuclear physicists have been pushing the boundaries of the periodic table, trying to find the optimal reactions for synthesizing new elements. Scientists from the National Centre for Nuclear Research (NCBJ) and the Faculty of Physics at the University of Warsaw have succeeded in creating a new theoretical model that describes the key stage in the production of the heaviest atomic nuclei.
Superheavy elements are synthesized through fusion reactions of the nuclei of two lighter elements. One of the methods for obtaining them is the so-called cold fusion, in which the accelerated in a cyclotron nuclei of a relatively light element collide with a target made of lead or bismuth. As a result of the collision of the two atomic nuclei, a strongly interacting system is formed which, with a certain probability known as the fusion probability, may combine. The atomic number of the resulting object is equal to the sum of the atomic numbers of the projectile and target nuclei. Such an object, immediately after formation, is „hot” – it has an excess of energy relative to its ground state. Therefore, it is not stable and very quickly tries to „cool down”, most often by decaying into smaller fragments or by emitting neutrons and gamma radiation. In the so-called cold fusion reactions, the energy excess is relatively low, and the resulting system cools down and reaches a final state after the emission of one or two neutrons. The new atomic nucleus formed in this way captures electrons within 10–14 seconds and forms an atom that is ready for further study.
The most challenging part of the entire synthesis process to investigate and describe is the stage where the two atomic nuclei merge into one object. Despite many years of research, there is no consensus among scientists on the mechanism of this process. Understanding this stage of the reaction is fundamental to the synthesis of new elements and the expansion of the periodic table. Therefore, scientists from the National Centre for Nuclear Research (NCBJ) and the Faculty of Physics at the University of Warsaw decided to create a theoretical model that explains the measured fusion probabilities and provides realistic predictions for synthesis reactions that have not yet been tested.
„During fusion, the shape of the system evolves from the configuration of two touching atomic nuclei to a single compact shape. The model we have created describes this process based on a random walk scheme in a multidimensional deformation space,” explains Aleksander Augustyn, a PhD student at NCBJ working on this issue.
„In the simulations we conducted, it turned out to be crucial to include the dipole variable and to describe the shape evolution not from the center of mass, but from a reference frame located at the contact point of the projectile and target nuclei. This allowed us to describe a wide class of shapes using a single parameterization,” explains dr Tomasz Cap, the first author of the article.
„In the simulation of the process, the system has full freedom of movement and can choose the most favorable paths leading to fusion. However, exploring the multidimensional space is numerically expensive. Calculations of this type were made possible by using a computing cluster at the Świerk Computing Centre,” adds Aleksander Augustyn.
„In the calculations, we also included quantum effects, which are essential for the existence of superheavy nuclei, and introduced the dependence on the angular momentum of the system. In non-central collisions of the projectile and target nuclei, rotational energy significantly reduces the chances of synthesis. Considering these factors improved the agreement of the calculations with the measurement results,” indicates Professor Krystyna Siwek Wilczyńska from the Faculty of Physics at the University of Warsaw, collaborating with the NCBJ team.
„The model is promising and efficient enough that we plan to apply it to analyze the fission process as well as conduct a more detailed analysis of shape evolution. We expect that soon we will be able to use it to describe processes such as fast, asymmetric fission at the beginning of fusion reactions. We also aim to enrich our theoretical description by including the possibility of neutron and light charged particle emission during the random walk in the multidimensional deformation space. Such an approach would allow for a simultaneous description of fusion and cooling processes, which has not yet been achieved,” summarizes dr hab. Michał Kowal, head of the Theory Department at NCBJ.
The paper has been published in the prestigious journal Physical Review C in the Letters section and is available at the following web address https://doi.org/10.1103/PhysRevC.109.L061603
Krzysztof Petelczyc