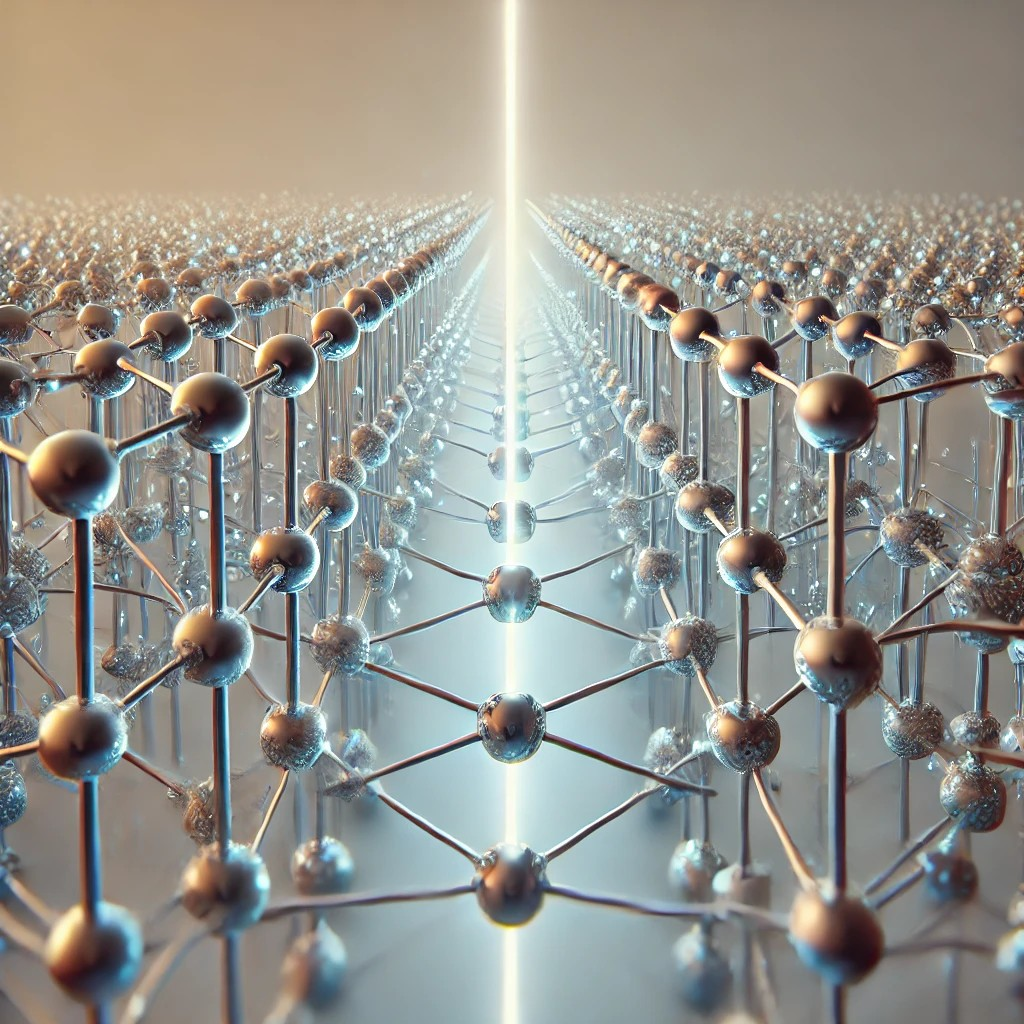
Research on radiation- and chemical-resistant semiconductors at the NCBJ Nuclear Microanalysis Lab
22-11-2024
Currently, materials research is driven by new technologies, resulting from the never-ending need to miniaturise devices and replace them with cheaper and more efficient counterparts. For several decades, new technologies have been based on semiconductor compounds, which are proving indispensable in optoelectronics, for the production of detectors, lasers, displays or LEDs, where the ever-present silicon cannot be used due to its intermediate and not very wide energy gap. At the NCBJ's Nuclear Microanalysis Lab, a group of researchers is currently working on using Ga2O3 gallium oxide for this purpose.
The history of semiconductor research by NCBJ scientists, initiated by Professor Andrzej Turos, has continued uninterrupted for almost half a century. This research was carried out mainly using the Rutherford Backscattering Spectrometry/Channeling (RBS/c) technique, which was the standard technique in our country thanks to the high-energy helium ion beam obtained in the Lech accelerator of the Van de Graaff type, located in the former premises of the NCBJ branch and the Faculty of Physics of the University of Warsaw at 69 Hoża Street. A natural consequence of this research was the development of a globally unique analytical tool for the analysis of RBS/c data, the McChasy programme. Today, research using the RBS/c technique is carried out thanks to numerous collaborations with European centres such as HZDR and the universities of Lisbon and Paris- Saclay. The McChasy programme is also constantly being developed and improved, making it more efficient, user-friendly and widely available.
Gallium oxide (Ga₂O₃) is a very popular semiconductor compound, which initially attracted the attention of scientists mainly due to its wide energy gap (~4.8 eV), the widest among other intensively studied materials such as ZnO, GaN and SiC. Ga2O3 is not a new material. Its history dates back about seven decades, but it remained in the background mainly because of the difficulty in producing high-quality crystals. It was only at the turn of the century that its true potential began to be realised due to its unique combination of characteristics: high electrical conductivity (7 MVcm-1 breakdown field), optical transparency, but above all because of its high resistance to radiation and chemical degradation. The story of gallium oxide is an example of how breakthrough technologies can emerge through a combination of patience, technological advances and interdisciplinary research. Although the material has long been overshadowed by more popular semiconductors, today it is one of the key candidates to revolutionise power electronics and optoelectronics.
Research on this material for optoelectronic applications by NCBJ scientists is conducted within the GaloRE project, led by NCBJ Professor Renata Ratajczak, PhD, and financed from NCN funds within the OPUS23 competition. The group's third paper this year has just been published, and more are planned. All results obtained so far in the project have been published in high-scoring journals from the Philadelphia list, such as Acta Materialia (200 points), Materials (140 points), or in a young journal from Nature's portfolio: Scientific Reports (140 points), which demonstrates both the relevance and high level of the research carried out.
The results presented in the publications concern the analysis of defects induced by ion bombardment. Such studies are extremely important from a practical point of view, as the resulting defects must be taken into account in the manufacture of micro- or optoelectronic devices operating under radiation exposure conditions, i.e. in outer space or in nuclear reactors. This is because the presence of defects significantly reduces certain material properties, e.g. electrical conductivity, luminous efficiency and effectively shortens the lifetime of devices built from the materials in question. In addition, the identification of defects is also very important, as it allows the selection of an appropriate method to eliminate the defects, e.g. by means of thermal annealing.
The authors believe that the research methodology they have developed with experience is the reason behind their success. This is because their experience shows that often a single research technique, e.g. RBS/c, even supported by advanced computer simulations, is not sufficient to uncover all aspects of the phenomenon under investigation. Each structural method has its limitations, and only a combination of multiple research methods is able to build a complete picture. The NCBJ team has been using a combination of RBS/c (Rutherford backscattering with channeling), XRD (X-ray diffraction) and TEM (transmission microscopy) methods for many years. "This unique approach to the study of this group of materials reveals further interesting aspects of the gallium oxide under study, resulting in further successes and recognition of our group in the industry," concludes NCBJ Professor Renata Ratajczak, PhD, head of the GaloRE project and first author of the latest publication.
She continues: "In the case of gallium oxide doped with ytterbium ions (Ga2O3:Yb), RBS/c studies have shown a very complicated process of radiation-induced defect accumulation, which has not been studied in such detail so far. The analyses showed that above a certain ion dose, the depth distribution of defects becomes two-humped. Moreover, defects from both areas showed a different sensitivity to further bombardment as well as annealing, suggesting that we are dealing with two different types of defects that give the same contribution to the channeling spectrum. XRD studies instead showed radiation-induced phase changes. However, this method cannot determine at what depth the new phase precipitates. Hence the need for TEM imaging. TEM studies require special preparation to be carried out on samples, which is why they are usually performed last. TEM imaging, carried out on a newly acquired and commissioned transmission microscopy device at NCBJ, confirmed the existence of two layers with different types of defects, and the use of an additional attachment of this microscope for the study of electron diffraction (SAED) made it possible to identify which layer is this newly formed radiation-induced γ-phase." The NCBJ group's research additionally showed that a radiation-induced amorphous phase appears in the layer closer to the surface. This discovery has reunited the previously divided scientific world. Some of the major scientific groups working on this issue reported that Ga2O3 crystals undergo amorphisation as a result of radiation, while others reported that the crystal instead becomes structurally self-ordered, switching to a new γ-phase. Researchers at the Nuclear Microanalysis Lab have resolved this dispute by showing that both groups are correct, as under certain conditions both phases exist simultaneously. Whether this is due to the ions used, the bombardment conditions or the dose, however, remains the subject of further research.
Additional information:
To study radiation-induced defects in materials with a crystalline structure, the RBS technique in ion channeling mode is commonly used. Such structure defects can already arise at the crystal growth stage, but the more interesting ones are mainly the result of interaction of the material with ions, e.g. in outer space or a nuclear reactor. The effects of irradiation under such conditions take years to develop, but in the laboratory the process can be quickened using a particle accelerator. Bombarding a material with high-energy ions is also a common method of intentionally introducing dopant ions into a crystal matrix, e.g. to change its optical properties, as is the case with Ga2O3 into which Yb atoms are introduced. Accelerated to high energy, the ions are directed to and penetrate the sample material, collide with the crystal atoms and can knock them out of their normal positions, thus losing energy and changing the direction of their motion. In turn, the atoms knocked out in this way can knock out the next atoms, creating a "disorder" in the crystal lattice. The situation is analogous to that which we can observe on a billiard table, where the first ball strikes a triangle of balls stacked on the table. In the case of intentional ion bombardment, however, we are dealing with 1015 such balls. This is, in simple terms, how defects in the crystal structure are formed. When there are a large number of defects, they tend to merge and transform into new, more complex forms. The resulting changes can be studied using various techniques, such as transmission microscopy (TEM), X-ray diffraction (XRD), or just ion channeling (RBS/c).
In the RBS/c technique, the material is again bombarded with ions accelerated to high energy, but in this case these are very light ions (usually helium). Unlike heavy ions, they bounce off the atoms of the crystal (we use the term scatter, as there is no physical collision but electrostatic repulsion) and the loss of energy gives information on which atom and at what depth the ion has scattered. If the crystal is properly orientated so that the accelerated ions travel through empty spaces between rows of atoms, known as channels (hence the name channeling), then scattering occurs very rarely. However, if there is a defect in the path of the ion, then the probability of scattering increases significantly. The shape of the collected RBS spectrum strongly depends on the type of defects the ion has encountered on its path. A natural consequence of such research was the development of a globally unique analytical tool for the analysis of RBS/c data, the McChasy programme, which allows signals from different types of defects to be separated.