International projects
The AKARI (ASTRO-F) satellite mission was launched on 2006 February 22, and carried out a series of infrared photometric surveys in the near- (NIR), mid- (MIR) and far-infrared (FIR) passbands until the satellite termination in 2011. During its operation, AKARI performed deep survey of the north ecliptic pole in NIR and MIR passbands, survey of south ecliptic pole in FIR and all-sky survey in MIR and FIR.
Results obtained by the AKARI satellite contributed a lot to our understanding of the dust astrophyscis and galaxy evolution. Currently AKARI provides one of the most complete and deep infrared survey data. The NCBJ group is involved in the AKARI project from the very beginning. Main fields of scientific interest of our group in the AKARI project are focused on galaxy evolution physics, observational cosmology and machine learning application.
Athena (Advanced Telescope for High Energy Astrophysics) is an X-ray mission accepted by the ESA to address the Hot and Energetic Universe science theme. The mission will be launched in 2030 and placed at the second Sun-Earth Lagrangian point (L2). The planned mission lifetime is five years, but the mission is expected to last longer. ATHENA will be equipped with two scientific instruments: the X-ray Integral Field Unit and the Wide Field Imager.
The X-ray Integral Field Unit (X-IFU) will provide spatially resolved high-resolution X-ray spectroscopy from 0.2 to 12 keV, with energy resolution of 2.5 eV for the energies lower than 7 keV (for higher energies E/dE=2800).
The WFI has a large field of view 40'x40', and very high angular resolution, 5''. It will observe in the energy range 0.2 -- 15 keV with resolution 170 eV at 7 keV. The planned time resolution for this instrument is 80 µsec. It's scientific goals are related to high energy phenomena, and include studying hot baryons in groups and clusters of galaxies, accretion processes onto compact objects, and GRBs and other transient objects.
Cherenkov Telescope Array (CTA) is an international project with a goal to construct ground-based astronomical observatories, designed to detect high-energy gamma rays using atmospheric blue-flash (Cherenkov light) imaging techniques. Two observatories are planned, one in each hemisphere of the Earth. Each will include several dozens of synchronized large optical telescopes. The Cherenkov light flashes recorded by them will allow to gather information about gamma-ray energies ranging from 20 GeV to over 300 TeV.
The CTA Consortium includes researchers from 32 countries, including Poland. The Polish Consortium of the Cherenkov Telescope Array Project is formed by 13 scientific institutions contributing to the project, including NCBJ. The activities of the Polish Consortium are in the area designing and manufacturing prototypes of equipment intended for application in CTA, including the small Cherenkov telescope SST-1M and new technology mirrors for medium size telescopes (MST). Poland provides also large computing and data storage resources, and contributes into a number of other activities of CTA.
The research carried out by the NCBJ researchers in preparation for the CFT data is covered by the grant of the Polish National Science Center UMO-2016/22/M/ST9/00583 led by the Jagiellonian University.
See also:
CTA webpage
The Einstein Telescope (ET) is the third-generation interferometric gravitational wave (GW) observatory proposed by leading European gravitational wave experimental research institutes coordinated by the European Gravitational Observatory. In order to reduce seismic noise and gravity gradient noise it will be located underground. Its arms are planned to be 10 km long arranged in an equilateral triangle with two detectors in each corner. Detectors at each corner would be composed of two interferometers: one optimised for frequencies below 30 Hz and one optimised for operation at higher frequencies. Sensitivity of the ET would be an order of magnitude better than LIGO/Virgo detectors, meaning that it would be able to probe three orders of magnitude greater volume of the Universe leading to an unprecedented statistics of GW sources originating at high redshifts. It will elevate gravitational wave physics from weekly or monthly detections to a high-statistics era allowing high-precision astronomy and confronting the General Theory of Relativity with a plethora of experimental tests.
In the department we are studying science cases for the ET focused on prospects for precision cosmology, detections of gravitationally lensed GW signals and testing gravity theories beyond General Relativity.
The Herschel Extragalactic Legacy Project (HELP) brings together a vast range of data frommany astronomical observatories. The key focus of the project is the Herschel Space Observatory data, which maps dust-obscured star formation over 1300 deg2. With this unprecedented combination of data sets, itis possible to investigate the evolution of galaxies across cosmic time, and how the star formation versus stellar mass relation (main sequence)of star-forming galaxies depends on the environment and many other aspects of galaxy evolution. HELP collates and homogenizes observations (λ from the radio to the UV) from many astronomical observatories, and moreover, estimates key physical parameters of observed galaxies.
The main goal of HELP is to remove the barriers to multi-λ statistical survey science and to produce value-added resources for astronomers to easily interpret the high redshift Universe. Understanding the evolution of galaxies across cosmic time is one of the great challenges of astrophysics. At the present day, galaxies found in different environments are very different from each other. To understand how this came to be we need to map a wide range of environments in the early Universe using telescopes that probe the different physical processes. Many astronomical facilities have thus been undertaking ambitious programmes to observe large areas of the distant Universe to study galaxy evolution and most of these complete in the next four years. HELP brings together key members of the various teams to combine these data homogenously.
PI of the project: S. Oliver (Sussex University, UK)
Acknowledgements:
The Herschel Extragalactic Legacy Project, (HELP), is a European Commission Research Executive Agency funded project under the SP1-Cooperation, Collaborative project, Small or medium-scale focused research project, FP7-SPACE-2013-1 scheme, Grant Agreement Number 607254.
„ASTROdust: a complete census of dust attenuation in galaxies based on the analysis of millions of galaxies observed by Herschel” is the project SONATA BIS 2018/30/E/ST9/00082 (founded by National Science Centre, Poland).
See also:
HELP webpage
Publications related to HELP
The National Centre for Nuclear Research is participating in LIGO-Virgo Science Collaboration devoted to research on gravitational waves since 2008. Head of gravitational wave research group is Prof. Andrzej Królak. The group is doing research on continuous gravitational wave signals and multi-messenger astronomy. The group have heavily contributed to first direct detection of gravitational waves (2015) and first observation of electromagnetic counterpart to gravitational wave transient event (2017).
See also:
LIGO Webpage
The Low-Frequency Array, or LOFAR, is a large radio telescope network located mainly in the Netherlands, completed in 2012 by ASTRON, the Netherlands Institute for Radio Astronomy and its international partners, and operated by ASTRON's radio observatory, of the Netherlands Organization for Scientific Research. LOFAR is currently the largest radio telescope operating at the lowest frequencies that can be observed from Earth. Unlike single-dish telescope, LOFAR is a multipurpose sensor network, with an innovative computer and network infrastructure that can handle extremely large data volumes.
BP4 contact person: dr hab. Katarzyna Małek
See also:
LOFAR webpage
Publications related to LOFAR
The European Space Agency's Planck satellite was a mission dedicated to studing the early Universe and its subsequent evolution by mapping the anisotropies in the cosmic microwave background (CMB) radiation. It was launched in May 2009 and scanned microwave and submillimetre sky until October 2013 producing high-resolution and high-sensitivity all-sky maps in nine frequency bands, from 30 to 857 GHz.
The main objective of the Planck mission was to measure the spatial anisotropies in the temperature of the CMB to an accuracy determined by fundamental astrophysical limits, by that obtaining virtually all the cosmological information encoded in the temperature anisotropies of the CMB. The satellite was also designed to measure to high accuracy the CMB polarisation anisotropies which provide not only a plenty of cosmological information but also yield a unique probe of the epoch of the Universe history when the first stars and galaxies formed. Lastly, Planck was the first submillimetre mission to map the entire sky to sub-Jansky sensitivity with resolution better than 10', thereby enabling to learn a wealth of information on the properties of extragalactic sources and on the dust and gas in our Galaxy.
See also:
PLANCK data products
POLAR-2 space instrument, currently in development, is devoted to measure polarisation of Gamma Ray Bursts (GRBs). It will be launch and placed aboard Chinese space station Tiangong-3 in 2024. Project is carried out in Swiss, Polish, Chinese and German collaboration. POLAR-2 is a successor to POLAR experiment that was placed aboard Chinese space station Tiangong-2.
Polish PI: prof. dr hab. Agnieszka Pollo (BP4)
The Transient Optical Robotic Observatory of the South (TOROS) project is devoted to search optical counterparts to gravitational wave transient events. TOROS project is being led by Center of Gravitational Wave Astronomy of The University of Texas Rio Grande Valley (Head PI: Prof. Mario Diaz), in cooperation with Institute of Theoretical and Experimental Astronomy at the University of Córdoba in Argentina. Other institutions that contribute are Texas A&M University and the National Centre for Nuclear Research. The National Centre for Nuclear Research is responsible for development AI methods for image processing.
Polish PI: dr Adam Zadrożny (BP4)
See also:
TOROS webpage
The “VIMOS Public Extragalactic Redshift Survey” (VIPERS) is an European Southern Observatory (ESO) Large Program that mapped the spatial distribution of galaxies over an unprecedented volume of Universe up to z~1. The project used the VIMOS spectrograph at the 8 m Very Large Telescope to measure the spectra for more than 90000 galaxies. Optimizing the combination of multi-band photometry with the multiplexing capability of VIMOS, VIPERS fills a unique niche in galaxy surveys at this redshift.
A color-color pre-selection allowed the survey to focus on the 0.5 < z < 1.2 redshift range, yielding an optimal combination of large volume and high effective spectroscopic sampling. VIPERS has produced a data set that in many respects represents for the first time the equivalent at z~1 of the large surveys of the "local" (z<0.2) Universe built at the beginning of this century like the Sloan Digital Sky Survey (SDSS) and the 2df Galaxy Redshift Survey (2dFGRS).
Our group collaborates internationally to expand and compare the study a low redshift to higher redshift with VIPERS data and the application of machine learning techniques in order to study how galaxies evolve.
See also:
VIPERS webpage
The VIMOS Ultra Deep Survey (VUDS) is a spectroscopic redshift survey of ~10.000 very faint galaxies selected using a combination of photometric redshifts and color properties. This allowed observations of galaxies at a very early stages of their formation and evolution – redshift range 2 < z ≲ 6. Additionally the survey covers big area of 1 deg2 separated between three independent fields: COSMOS, ESDFS and VVDS-02h – mitigating cosmic variance effects.
This unprecedented large high-redshift spectroscopic survey allows to accurately study:
- The evolution of the global star formation rate and build up of the mass function for galaxies with different properties.
- Clustering of galaxies and the mass growth of underlying dark matter halos at the early stages of the universe evolution
- Contribution of mergers to the mass growth of galaxies
- Properties of a very young galaxies
- and many more
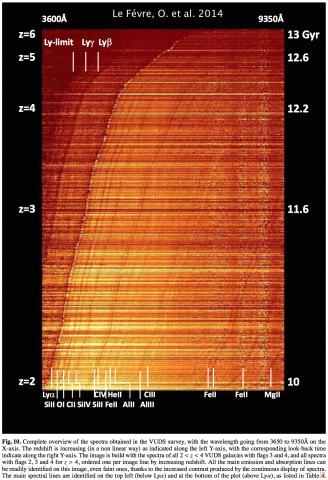
In BP4 department Anna Durkalec use VUDS survey to study galaxy clustering in the Halo Occupation Distribution model (HOD) framework.
See also:
VUDS webpage
Vera C. Rubin Observatory (formerly Large Synoptic Survey Telescope, LSST) is a project whose aim is to built an 8.4 m telescope capable to produce deep wide synoptic surveys of all sky, in particular to conduct the Legacy Survey of Space and Time (LSST). Its first light is expected in 2021. The summit facility is located on the Cerro Pachón ridge in north-central Chile. LSST leverages innovative technology in all subsystems, in particular the camera (3200 megapixels, the world’s largest digital camera) and telescope (simultaneous casting of the primary and tertiary mirrors; two aspherical optical surfaces on one substrate), as well as data management (15 terabytes of data nightly, nearly instant alerts issued for objects that change in position or brightness). The 8.4-meter telescope uses a special three-mirror design, creating an exceptionally wide field of view, and has the ability to survey the entire available sky in only three nights.
The goal of the Rubin Observatory is to conduct a 10-year survey of the sky that will deliver a 200 petabyte set of images and data products related to 37 billion astronomical sources. They will allow to address some of the most pressing questions about the structure and evolution of the universe and the objects in it. LSST will focus on four main science areas: (1) nature of Dark Matter and Dark Energy; (2) hazardous asteroids and the remote Solar System; (3) the transient optical sky; (4) the formation and structure of the Milky Way.
Rubin Observatory will conduct a deep survey over an unprecedentedly big area of sky - the main survey will cover 18 000 square degrees. Frequency of observations will enable images of every part of the visible sky to be obtained every few nights. This operation mode executed for at least ten years will allow to achieve astronomical catalogs thousands of times larger than have ever previously been compiled.
The Polish LSST-PL consortium presently consists of 20 affiliated members from six Polish institutions: NCBJ (leader of the consortium), Jagiellonian University, Warsaw University, Nicolaus Copernicus University in Toruń, Nicolaus Copernicus Astronomical Center of the Polish Academy of Sciences, Center for Theoretical Physics of the Polish Academy of Sciences. The Polish participation in the project us funded by the grant of the Polish Ministry of Science and Higher Education DIR/WK/2018/12.
The research carried out by the NCBJ researchers in preparation for the coming LSST data is partially covered by grants of the Polish National Science Center: UMO-2018/30/E/ST9/00082 and UMO-2018/30/M/ST9/00757.
See also:
Vera C. Rubin Observatory webpage
Internal projects
SONATA BIS 2018/30/E/ST9/00082 (funded by National Science Centre, Poland)
Stars are seldom found alone in the universe. They are formed and live out their lives together with other stars in massive associations known as galaxies. Optical telescopes show that our Universe if full of such islands. But the visible light is not the best source of information about galaxies and the structures inside them. Massive stars can emit in visible wavelengths such amount of energy that they seem to be disproportionally more significant than they really are. In the infrared this imbalance is less extreme, so we can get a more representative view of how the structure of the galaxy looks like, and how stars are actually distributed. Additionally, many galaxies are also filled with dust clouds that obscure our view of the overall structure of the galaxy. But thanks to the characteristic of dust, which become more transparent towards longer wavelengths, and is some ranges – it re-emits the ultraviolet light emits by young stars, galaxies observed in infrared light shows all the inaccessible in visible light structures of the galaxy. All those reasons cause that observation of galaxies in infrared range allows uncovering of areas inaccessible for optical observations.
The main aim of the project is to statistically investigate the attenuation of dust in galaxies and its dependence on the types of galaxies in different space ages. We will use a unique sample of one million galaxies observed in the infrared by the Herschel satellite. We plan to construct the correct attenuation curves for different types of galaxies and to examine how they have evolved over the past 10 billion years. This will lead to a re-evaluation of the basic physical properties of star-forming galaxies and will provide new tools to astronomers who occupy themselves with these fascinating objects.
MAESTRO 2023/50/A/ST9/00579 (funded by National Science Centre, Poland)
PI: prof. dr hab. Agnieszka Pollo
All existing surveys of astronomical objects have limitations, due to the accuracy of the instruments and, in the case of ground-based observations, also to the fact that the Earth's atmosphere interferes with our observations. Consequently, our knowledge of the Universe is mainly based on observations of relatively bright objects.
Meanwhile, we have known since the 1980s that there are galaxies in the Universe that very easily escape observation. These are so-called low surface brightness galaxies, or more precisely, galaxies with a brightness lower than the typical brightness of the night sky. The first such galaxy was discovered by astronomer David Malin in 1986 - it is called Malin 1 and remains one of the largest known spiral galaxies to this day. Most of the currently known galaxies of this type are much smaller dwarf galaxies, but the variety of types among these galaxies is similar to that of their bright cousins.
The number of known low surface brightness galaxies has been increasing over the years. Initially, they were discovered mainly by chance, on the occasion of deep observations of selected areas of the sky. A systematic search for these objects has only recently begun. It turned out that they are virtually everywhere. Also, bright galaxies are surrounded by a faint glow, which sometimes extends over enormous distances.
There are still more questions about these objects than answers. How many are there? How much 'normal' baryonic matter is in them, and can they help explain the so-called 'missing baryons' puzzle - the fact that we observe less baryonic matter in the Universe today than in earlier cosmological epochs? Can they explain another cosmological problem - the so-called problem of missing satellites, i.e. the fact that we observe fewer small galaxies than predicted by theoretical models? What processes led to their formation, and why are they different from 'normal' galaxies? When did such galaxies form, and what did they look like in the past?
The atmosphere has been heated by studies of so-called ultra diffuse galaxies, a particular subtype of low surface brightness galaxies found in galaxy clusters. Among these, galaxies have been found that consist almost entirely - 99% - of dark matter, as well as galaxies that appear to be completely devoid of dark matter. How is this possible and what processes lead to such extreme objects? Can their existence be explained on the basis of the currently accepted cosmological model? Or do we need to change the laws of physics for them?
Answering these questions requires observing large areas of the sky with large telescopes capable of observing the faint glow of low surface brightness galaxies. A revolution in sky observation will be brought about by the Very Rubin Observatory, whose construction in Chile is just finishing and whose main objective is to create the Legacy Survey of Space and Time (LSST). The 8.4-metre telescope installed there, equipped with the world's largest wide-angle camera, will start scouring the entire southern sky in two years' time.
The aim of the proposed project is to build a team that, using simulations and currently available data, will develop new and improved methods to search for and classify low surface brightness galaxies, improve and develop new methods to study their physical properties, and finally, after applying the developed methods to the LSST data, try to answer questions about the role of low surface brightness galaxies in the evolution of the Universe - and the relevance to the laws of physics that govern it.
SONATA BIS 2020/38/E/ST9/00077 (funded by National Science Centre, Poland)
PI: dr Ambra Nanni
Just after the Big Bang, the Universe was only made up of hydrogen and helium.
We need to wait for the birth of the first stars to witness the appearance of heavier elements (metals) which can only be synthesised in their interiors, where temperatures reach tens of millions degrees. Metals are later released in the interstellar medium at the end of stellar evolution and new generations of stars are then formed from the metal-enriched gas. This process is known as the life-cycle of metals in galaxies. Metals are only the 2% of all the visible matter but they give origin to almost the totality of what we see on Earth, including living beings.
During their evolution stars can lose a substantial fraction of their mass, developing a dense and extended circumstellar envelope around them. This is one of the main sites for the formation of small solid particles starting from the available metals, known as "dust grains". In space, about half of the metals are condensed in dust. Despite dust being a small fraction (1%) of the total visible matter, it plays a crucial role in the evolution of the objects populating the Universe, including the formation of stars and planets that could harbour life.
The only way we have to study the properties of dust around stars and in galaxies is by investigating its effect on the radiation of stars. Indeed, dust grains absorb stellar radiation, heat up and re-emit this energy at longer wavelengths. Thus, the overall effect of this process is to modify the radiation originally emitted by stars and, consequently, the radiation that we receive and that we study. This effect is clearly shown in figure 1: in the upper panel we see the Milky Way in the visible band. The presence of dust clouds in the Galactic plane absorb the light from the stars and originates some "black spots". In the lower panel the Milky Way is shown in the infrared bands where dust re-emits the absorbed energy. We therefore see a bright emission at the Galactic plane where we have dust. By employing reliable theoretical models of the light emitted by stars and reprocessed by dust, it is possible to derive the physical properties of galaxies and to study how dust evolves from the beginning of the Universe up to the present days.
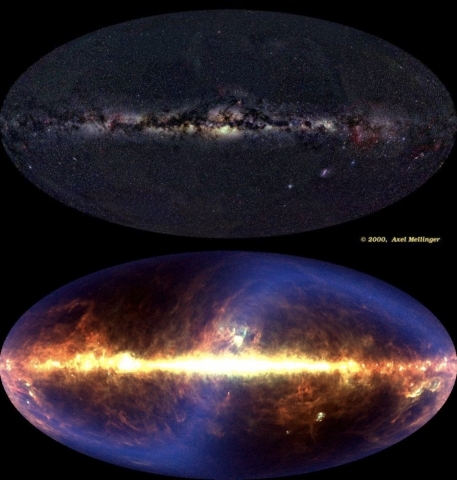
Figure 1: Upper panel: view of the Milky Way in the optical where stars emit: the black spots in correspondence of the galactic disc are due to dust clouds which absorb the radiation from the stars behind them (credits: Axel Mellinger). Lower panel: view of the Milky Way in the infrared bands where the light absorbed from the stars is re-emitted by the dust grains in the clouds (credits: COBE satellite)
The project DINGLE aims to model dust formation around mass-losing stars and in the interstellar medium of galaxies, and to develop a reliable description of light absorption and re-emission from dust grains. The aim is to study dust in different galaxies and compare our theoretical models with their observations.
The project will increase our understanding of the life-cycle of metals and dust from the first galaxies formed to the present day.
SONATA 2020/39/D/ST9/00720 (funded by National Science Centre, Poland)
Galaxies in the Universe are complex systems that consist of gas, stars, metals and dust. The nature of galaxies is not only affected by the interplay of these baryonic components, but they also share a strong relation with surrounding halos of invisible, dark matter, into which they are embedded. As galaxy progressively ages since it’s formation, it passes through multiple evolutionary stages. Understanding physical mechanisms behind this evolution is one of the main goals of modern astronomy.
It has long been postulated that dust plays vital role in evolution of galaxies and their stars. It induces the formation of molecular clouds, supporting the birth of new stars. Dust grains also absorb stellar ultraviolet light at shorter wavelengths and re-emit it into in infrared, dramatically affecting the observed galaxy spectra. Major advances in far-infrared instrumentation in recent years, both space-based and ground-based, has led to the detection of more than a million, so-called dusty star-forming galaxies, very distant and massive objects that form large amount of dust and young stars. These discoveries posses a serious challenge to astronomy: many of these dusty ’Giants’ have been formed when the Universe was very young, sometimes even less than 1 billion years after the Big Bang, and we wonder how could such large amount of stars and dust have been produced so early in time?
Many theoretical models postulate that nature of dusty star-forming galaxies is key to understand formation of most massive structures in the Universe. The reason: these are thought to be direct evolutionary link between the most distant, active star-forming galaxies, and the most massive elliptical galaxies that reside in huge clusters we see in the local Universe. Probing and understanding this connection is a key task for modern infrared astronomy, but it is also highly challenging and under-investigated.
The project Dusty Giants aims at understanding the role of dust in galaxies and their large scale environments in galaxy evolution. The Project will pioneer the the methods to investigate the link between galaxy dark matter halos and life cycle of dust. This will be achieved by combining observations and state-of-the-art simulations in order to quantify how the relative ratio between dust and stars changes in different galaxy populations over cosmic time.
One of the expected outcomes will be a new technique of selecting and understanding distant proto-clusters. The Project is thus timely for providing the solid ground needed for interpreting future unprecedented data sets of large scale surveys of galaxies in the distant Universe (e.g. Euclid mission, Vera C. Rubin observatory) and their synergy with current millimetre telescopes on Earth (NIKA2, ALMA).
PRELUDIUM 2023/49/N/ST9/00746 (funded by National Science Centre, Poland)
Starting from baryonic matter, including stars, dust, and gas, through mysterious dark matter, to gravitational and electromagnetic interactions, galaxies encompass a vast majority of the physical processes known to modern science. Simultaneously, they are a crucial aspect of contemporary astronomy due to their relation to the evolution of the entire Universe. For this reason, investigating their formation, evolution, and the circulation of matter within these systems is of fundamental importance.
Observations confirmed by theoretical models show that dust and the entire interstellar medium accumulated within a galaxy are essential factors shaping its evolution. From the formation of molecular clouds to the significant influence on star formation and the absorption of high-energy electromagnetic radiation, galaxies as we know them could not exist without dust. However, modelling and studying galaxies based on observations are challenging due to the mentioned absorption of ultraviolet light by dust. By absorbing a significant portion of the radiation produced by young and massive stars and then reemitting it in the infrared, dust shifts the energy spectrum of the galaxy towards longer wavelengths. Until recently, studying the interstellar medium, including dust, in the young Universe was very challenging due to insufficient infrastructure. However, the recent launch of the James Webb Space Telescope may shed new light on these previously unnoticed infrared-quiet galaxies.
Until recently, it was believed that the majority of these passive galaxies were elliptical. However, recent analyses indicate that galaxies in the young Universe may have looked and behaved slightly differently than previously assumed. Therefore, conducting large surveys using state-of-the-art instruments from the aforementioned space telescope can shed new light on many aspects of galaxy evolution. This ranges from the dust content in the interstellar medium in the early Universe to the relationships between star-forming activity and morphology, as well as the influence of the environment on galaxy evolution.
In this project, we intend to utilize the latest data from the James Webb Space Telescope, which, in conjunction with other observations, including those from the Hubble Space Telescope, will help us understand the global dependencies of galaxy and interstellar medium evolution, particularly with regards to dust. Our goal is to uncover the origins of galaxies as they exist today.
OPUS 2020/37/B/ST9/01821 (funded by National Science Centre, Poland)
The research project “Search for macroscopic dark matter by the ground-based and space-based high sensitivity cameras” aims at discovery of mysterious dark matter. Already in the 19th Century, scientists recognized “something” filling in Our Universe even though they are not visible to our eyes. Last fifty years, we are more confident “they exist”. Moreover, recent observations indicate no more than 4% of Our Universe is visible. So far, many efforts were made to discover dark matter, however, no successful results have been report.
In this project, we try the never tried new approach to search for such mysterious dark matter. In 1984, De Rujúla and Glashow published an attractive theory on the Nature journal. They postulated a new type of dark matter called “nuclearite”. Interestingly, they demonstrated nuclearites can be “observed” if they exist and pass in the atmosphere. Nuclearites may be even seen like shooting star meteors. Nuclearites are a type of dark matter particle called “strange quark matter”. Like ordinary element like carbon, oxygen, iron, etc., they are made of elementary particles called quarks which are as very dense as 360,000,000,000,000 (= 360 trillion) times heavier than water of the same volume. Nuclearites may have any mass. Theoretically, it can be as heavier than the Sun and as big as ~20 km quark star like already discovered compact stars called “neutron stars”.
Copernicus proposed Earth revolving the Sun in the 16th Century. Now, we know the Sun is not the center of the Universe. It revolves around Our Milky Way Galaxy at ~27,000 light years from the center of the Galaxy. Along with Sun, we move the toward the direction of the Cygnus constellation at about 250 km/s. Scientists cannot explain such a high speed without dark matter. Nuclearites have a mass and are bound by the Milky Way gravity. Near the Sun, they also move at similar speed to any direction if nuclearites exist. From the observers on the Earth, they look a bright light spot with a speed up to ~500 km/s.
The model of De Rujúla and Glashow indicates 1 kg nuclearites has a ~600 nm size. Very fast moving nuclearites hit the atoms and heat these atoms to result in the well-known black-body radiation emitting light until they cool down. This phenomenon takes place along the all path of the nuclearite. This light emission is similar to meteor shooting stars but happens near the sea level where air density is high. The speed is also different from meteors. The source of the meteors is considered the dust of comets revolving the Sun like planet, i.e. they are bound the Sun's gravity. The speed limit of meteors is no faster than 72 km/s to our eyes on the Earth. To find the nuclearites, we have to be able to take the video of even faster speed flying objects.
In this project, we are interested in nuclearites by two experiments. One is Mini-EUSO and the other is DIMS. We started the operation of Mini-EUSO a 25 cm telescope on the International Space Station (ISS) in October 2019 to monitor the Earth in the nighttime in the ultra-violet band. DIMS operates ultra-high sensitivity digital cameras for the meteor observation in the West Utah Desert, USA. DIMS group plans to build four automatic observation camera units deployed for meteors, particularly those with the origin out of the Solar System. This project will add another unit to specialize the nuclearite search.
The Mini-EUSO telescope is able to make a ultra-fast video at maximum 400,000 frame per second for 2000 pixels to study in details about the lighting and other phenomena. In orbit, Mini-EUSO keeps taking chronophotographic images about 24 frames every second to makes videos of 300 km x 300 km area. We already succeeded in making a first image of central Poland in the UV band. Many meteors were also observed. Seen from 400 km orbit, “500 km/s” looks slow enough by analyzing the Mini-EUSO data to search for kilogram nuclearites.
DIMS uses commercial digital cameras but with specially high sensitivity CMOS (Complementary Metal-Oxide-Semiconductor) sensor. These cameras make it possible to take ultra-fast nuclearite near the ground from the first time since the 1984 article. Even with only 1/30 s exposure, stars of the magnitude 10 can be recoginzed. In the test in Utah, we found ~3800 meteors in 40 hours by using two CMOS cameras. These cameras are able to take a video at ~60 frames per second with 2 mega-pixels. We aim at searching for about 1 gram nuclearite passing within ~30 km in this project.
In this project, if we find something consistent with the nuclearite, it may be the first long awaiting discovery of dark matter. Even no discovery is made, we will be still able to claim nuclearites would not exist no more than a particular value depending on how long we can monitor the night sky. We also expect the future application of CMOS technology in the space. Stay tuned and thank you for your support to our project!
SONTATA 2022/47/D/ST9/00419 (funded by National Science Centre, Poland)
PI: dr Miguel Figueira Sebastiao
Galaxies that can be seen through amateur telescopes or on popular science images seem to be made of three components: an abundant quantity of stars and planets rotating around a central black hole. Nonetheless, the major part of galaxies is made of dust and gas at a temperature close to the absolute zero (−273 °C) and invisible to our eyes. Gas and dust tend to gather into huge clouds which are the birthplace of stars. For instance, one of the most popular stellar nurseries consists of three gigantic column of dust known as ”the Pillars of Creation”, and became famous thanks to the Hubble Space Telescope (and lastly with JWST).
When astrophysicists study the stars in our Galaxy, they have to distinguish between low-mass and high-mass stars because their formation, evolution and death are completely different. In particular, massive stars are able, at the very beginning of their formation, to sweep-up their surroundings thanks to powerful stellar winds and to the huge quantity of ultraviolet photons they emit. This phenomenon creates a layer of material around the massive stars and the whole structure is commonly called a ”bubble”. What is very interesting with these bubbles is that the surrounding dense layer of material is an excellent stellar nursery where a lot of massive newborn stars can be observed.
In my research project, I propose to observe these bubbles to study their properties and understand if they can efficiently form massive stars. Since the dust and gas are invisible to our eyes, we have to use specific telescopes dedicated to the observations of the coldest regions of our Galaxy. Using the data from the Herschel space satellite, I will study several bubbles, extract all the clumps of dust and gas found at their edges, and evaluate if these bubbles have the potential to form massive stars.
I will also observe the smallest structures inside these clumps thanks to very detailed observations from the ALMA interferometer, a system of 66 antennas working together to mimic an unique telescope of more than 1 km of diameter. I will also study the magnetic field with specific instruments able to detect the polarization of the light. These observations will tell us how the layer of dust and gas found around the bubbles is fragmenting, what physical processes govern this fragmentation mechanism and how massive stars are forming by unveiling the early beginning of their evolution. We will also compare bubbles in the Milky Way with bubbles in other galaxies to broaden our knowledge on the star-formation process.
This project aims at a better understanding of the role of bubbles in the formation of high-mass stars in our Galaxy. This is particularly important because thousands of bubbles are present in all galaxies and their crucial role in promoting the formation of a new generation of massive stars may change our view of the Universe’s history. Therefore, it is crucial to understand their role in the star-formation process in the global framework of galaxy evolution. Last but not least, due to the huge development of interferometry and the still unsolved question of high-mass star formation, I want, through this project, to promote the place of the National Centre for Nuclear Research (NCBJ), with a new Polish Community of students and researchers, to make it at the forefront of this promising research field.
PRELUDIUM 2022/45/N/ST9/01336 (funded by National Science Centre, Poland)
In the last two decades, astronomers have gained massive knowledge of how galaxies form and evolve. This advancement in the field of extragalactic astronomy was coupled with the rapid development of the instrumentation aspect of astronomy, which led to accumulating a large amount of observations of galaxies at different epochs of the lifetime of the Universe. With giant multiwavelength datasets, we realized that galaxies are not just entities in space and time. They are more like living organisms: They are born, they get old and then they die! Such evolution of a lifetime of galaxies is governed by the amount by which they form stars.
The vital organ of galaxies is their interstellar medium, where the building blocks of future stars are found. In the interstellar medium, dust plays a major role in the life cycle of galaxies, it shields the molecular clouds from hot radiations, promoting new stars to be formed. However, dust absorbs a big amount of the ultraviolet photons that originate in the young stars, and emits them in the infrared thermally. This effect is known as dust attenuation.
Galaxies emit across the full electromagnetic spectrum, and every wavelength range reveals to us information about underlying physical and chemical mechanisms. Ultraviolet photons give us information about the rate at which galaxies are forming stars, since newly-born stars emit predominantly in the ultraviolet range. Older stars shine their light in the visible range, and interstellar dust grains emit in the infrared. Detected fluxes across these wavelengths help us to construct spectral models of these galaxies. To model galaxies’ emission, it is essential to account for dust attenuation, by using an attenuation law
Different attenuation laws exist, but they are not universal and we still lack the knowledge of how to correctly attenuate the stars in different types of galaxies to reproduce their observed spectra. Not knowing the exact shape of the attenuation restricts us from correctly interpreting fundamental properties of galaxies, such as their age, mass and the rate at which they are forming stars.
Years worth of observations provided us with unprecedented high quality detections of millions of galaxies at different epochs of the Universe. With telescopes that observe across all the wavelength ranges, we are now able to study and analyze galaxies’ spectra to better constrain the properties of interstellar dust, and therefore understand the nature of dust attenuation laws and how they vary in galaxies with different spectral properties, various morphologies and that are located in different environments. The various wavelengths through which these galaxies are detected will help us in modeling the total spectrum coming from them. This in turn will allow us to constrain the physical properties of these galaxies, and to understand what is the role of dust in their interstellar medium.
In this project, I will statistically investigate dust attenuation in galaxies at different epochs of the Universe, to better understand the role of dust attenuation in the context of galaxy evolution, and to better estimate physical properties of galaxies. This motivates the quest to understand how galaxies form, and what is the role of dust in converting interstellar gas efficiently into stars.
SONATA 2023 2023/51/D/ST9/00147 (funded by National Science Centre, Poland)
Galaxies, large structures of stars, dust, and gas, do not live in isolation but tend to find oneanother and collide. During these collisions, the stars, dust, and gas in the galaxies are forced to move around violently, forming the beautiful structures we see in images of galaxy mergers. This motion of material can cause more stars to be formed at a much higher rate than would otherwise happen. This star-formation also has implications for the amount of dust and metals that are present in the galaxies, and how the dust obscures light. However, we do not have a solid idea of how the galaxy mergers change the amount of dust and metal present in a galaxy, or how the dust attenuates emission from the stars. To add to this, finding galaxy mergers is a non-trivial endeavour.
This project will investigate how we can more reliably identify galaxy mergers using their morphologies and how mergers influence their dust content, dust attenuation, and metallicity. This will be done by combining artificial intelligence (AI) with more traditional methods to detect galaxy mergers as well as use state of the art observations and techniques to study the galaxies' dust and metal.
For detecting galaxy mergers, this project will use images and morphological data of known galaxy mergers to compare AI results with results using morphology. It will continue to create new morphological parameters that can function with a similar ability to AI, assuming that AI works the best in the first experiment. This project will also use data from large simulations of our universe to train an AI to identify galaxies that are about to merge (pre-mergers) and have just collided with another galaxy (post-mergers).
Parallel to this, the project will study the dust content of galaxy mergers and compare it to the dust found in non-merging galaxies. This will use state of the art tools to extract the best information form state of the art data in the infrared, a key component for dust mass estimation. These data will also be used to help better understand how this dust hides optical light in merging galaxies compared to non-merging systems. These dust studies will be done for merging galaxies as well as pre-merging and post-merging galaxies, once the AI to detect these two merger stages has been finalised.
The project will also look at the metallicity of merging galaxies compared to non-merging galaxies. This will help us understand how galaxy interactions influence the amount of heavier elements present in galaxies.
With highly accessible techniques that will be developed in this project, the modern technologies used, and modern and near-future data sets, this work will be highly impactful and will influence future galaxy merger research.
Previous internal projects
SONATA 2013/09/D/ST9/04030 (funded by National Science Centre, Poland)
PI: dr Katarzyna Małek
FUGA 2015/16/S/ST9/00438 (funded by National Science Centre, Poland)
PI: dr Aleksandra Solarz
OPUS 2012/07/B/ST9/04425 (funded by National Science Centre, Poland)
PI: dr hab. Agnieszka Pollo
PRELUDIUM 2015/19/N/ST9/03708 (funded by National Science Centre, Poland)
PI: mgr inż. Zbigniew Plebaniak
The group of scientists from the National Centre for Nuclear Research are participating in the international satellite experiment JEM-EUSO (Japanese Experimental Module – Extreme Universe Space Observatory). The main JEM-EUSO objective are measurements of the ultra high energy cosmic rays (UHECR). UHECR are extremely energetic particles which come from the Universe. Their arrival direction distribution is nearly isotropic, as measured so far. The JEMEUSO detector is very fast camera (400 000 frames/sec) which would be placed at the International Space Station (ISS), and observe the Earth atmosphere from about 400 km. It will measure Extensive Air Showers (EAS) which are huge cascades of particles (and anti-particles) generated by the primary cosmic ray particle of very high energy in collisions with atmosphere nuclei. The cascade is moving in the atmosphere with the speed nearly to the speed of light in vacuum. The high speed camera is required to measure step by step time development of the cascade. The single measurement will allow to determine primary particle energy and direction. Many measurements (we expect to measure about 1000 particles with energies above 3×1019 eV) shall allow for search for astronomical sources of these particles, since their trajectories (if they are protons) shall be only slightly bended in cosmic magnetic fields. We expect to be able to confirm GZK cut-off, as well as measure events with still higher energies (like from „local” sources within GZK radius). JEMEUSO has also large scientific program for atmospheric phenomena measurements. In the JEM-EUSO project there are a few test projects. One of them is EUSO-TA experiment with objectives to calibrate the detector module and ground measurements of UHECR from the Telescope Array (TA) detector side (in Utah, USA), and with coincidence with TA.
Telescope Array is the largest cosmic ray experiment in the Northern Hemisphere. In the Utah desert it is far from any human made light sources, and it measures huge EAS during Moonless nights.
The main tasks of this project are calibration of EUSO-TA detector by set-up of many parameters of the camera to enable measurements of EAS, and measurement of EAS with EUSOTA working in coincidence with the Telescope Array experiment. The EUSO-TA detector is a single photo detection module (PDM) made of 36 photomultipliers (each with 64 anodes/pixels), similar to used or plan to use in another test experiments EUSO-Balloon, SPB-EUSO and Mini-EUSO.
Calibration can be made in a way to enable measurement and identification of stars which are in the field of view during the night measurements. With know brightness these stars are the reference objects for EAS measurements
During earlier measurements I have participated we had observed stars, air planes, lightnings, and several events which were probably EAS detections (it was the first test run of the EUSO-TA).
Elaboration of the automatic method for search for “cosmic ray” events in the hundreds of millions registered frames is part of this project, as well.
Within this project I have a plan to participate in measurements (shifts) in Utah. During these shifts I expect to observe EAS events with primary particle energies above 1018 eV. Events found and recognised as EAS would be compared with results of EAS simulations performed by other JEM-EUSO Collaboration members, and results will be published in the Experimental Astronomy (most likely). I am going to publish a paper with would describe performance of the high voltage power supply unit (HVPS) design and made in the Łódź Cosmic Ray Laboratory of the Astrophysics Department of NCBJ, where I am a PhD student.
The EUSO-TA detector has better angular resolution and faster camera than fluorescence detectors of the Telescope Array experiment. In this sense the EUSO-TA results are interesting complement of TA data base.
Realisation of this project would be important step towards the bigger projects like participation in experiments and data analysis from Mini-EUSO, SPB-EUSO and finaly in JEMEUSO. All these experiments are directed to measure and study of still poorly know nature of cosmic ray origin.
OPUS 2020/37/B/ST9/00466 (funded by National Science Centre, Poland)
PI: dr William Pearson
Galaxies, large structures of stars, dust and gas, do not live in isolation and are known to collide with one another. During these collisions, the galaxies become disrupted as the stars, dust and gas are moved around by the tidal forces created during these events. This movement of material can cause the gas to be compacted, resulting in periods of highly enhanced star-formation rates, know as starbursts. There is a wealth of observational and simulation information that shows that starburst galaxies are typically merging galaxies. However, what the observations cannot tell us is when these starbursts occur during a merger: are they early in the merger sequence, at the very end or somewhere in between. As a merger takes well over a billion years to complete, it is not feasible to watch a merger from start to finish to answer this question. On the other hand, simulations are able to see this but may not completely and accurately reflect what goes on in the real universe.
This project will determine how the star-formation rate of a galaxy changes as a merger progresses using observations. This is something that has not been done before and we have only recently developed the techniques needed to undertake such a study. While it is not possible to watch a single galaxy through an entire merger, it is possible to create a large sample of galaxies at different merger stages. However, identifying the merger stage is difficult, with only a simple pre-merger or post-merger classification having been done in the last year. This project will use the latest advances in artificial intelligence (AI) to identify the time before or after a merger event for a statistically large sample of galaxies. From this, an observed merger sequence will be determined and we will, for the first time, be able to see how star-formation rates in galaxies change as they merge and when these changes occur.
For this, we can use simulations of galaxy mergers. While not a perfect reproduction of the Universe, cosmological simulations are able to accurately reproduce the shapes of galaxies and how interactions distort these shapes. The simulations also provide a known time before or after a merger event. Thus, using images from simulations with known times before or after a merger event, an AI will be trained to be able to accurately determine the time before or after a merger happens. This AI will then be applied to thousands of images of real galaxies, providing accurate estimates of how long it is until a merger or how long it has been since the galaxies merged. The star-formation rates from these galaxies will then be determined and all the galaxies will be used to generate a statistical picture of how the star-formation rate of galaxies changes during a galaxy merger. From this, we will better understand why the universe looks like it does today.
The results of this work will provide a catalogue of merger times for use by other astronomers. Other physical properties of galaxies are believed to be changed as they undergo a merger and this catalogue will allow other groups to study these different phenomena, or find new properties that were not previously understood to be influenced by a galaxy merger. The AI will also be released, allowing other astronomers, and the wider public, to use and adapt it for their own needs.
ETIUDA 2016/20/T/ST9/00589 (funded by National Science Centre, Poland)
PI: dr Zbigniew Plebaniak
OPUS 2017/27/B/ST9/02162 (funded by National Science Centre, Poland)
PI: dr Jacek Szabelski
We want to measure and explore cosmic phenomena of extremely high energies: cosmic rays of ultra high energy. We do not know what objects emit so much radiation, nor how they do it. That is why we want to measure them with new satellite techniques. We participate in two big international scientific Collaboration JEM-EUSO. We have built high voltage power supply systems for all EUSO test experiments
Cosmic rays are energetic and stable particles and atomic nuclei coming from outside our Solar System. The most energetic ones are extremely rare. We observe them using the Earth's atmosphere as a target in which these particles interact and generate cascades of secondary particles, the number of which exceeds billions. We measure these cascades. The biggest experiment - Pierre Auger Observatory - has detectors on an area of about 3000 km2 and observes about 1 cascade of ultra-high energy once every 2-3 weeks. The particles that generate such cascades have energies several million times greater than energy of each proton currently accelerated at CERN in a supercomplicated accelerator. To measure even more of these cosmic ray particles, we want to build a detector - a space telescope that will observe much larger part of the atmosphere from the International Space Station. This will be a complicated, big, heavy and expensive experiment (EUSO). For now, we have built a few small experiments: two for balloons, one in the desert next to the second largest cascade detector - the Telescope Array and one small test experiment for the Space Station. We will analyze the data and build more detectors.
By the way, we will measure solar flares (also very energetic phenomena) and lightning and so called ionospheric discharges (most energetic phenomena in the atmosphere).
The main scientific task of this project is analysis of the data that has been obtained and will be obtained during the project. We also plan to continue our commitment to building subsequent experiments.
SONATA 2015/17/D/ST9/02121 (funded by National Science Centre, Poland)
PI: dr Anna Durkalec
Cosmology by its definition is the study of the origin, evolution and eventual fate of the universe. However, there is a lot of unknowns about the beginning of the universe, only uncertain theories about its future, and some huge questions about what happens in the middle. One of the most important of these questions is how the complex structure of galaxies observed in the local universe formed and evolved through time.
According to the modern cosmological models, which describes the state of our current understanding of the shape and evolution of the universe, the vast majority of its structure is build of something we can't directly observe. Because of that we named this component the ’dark’ matter, in opposite to the ’luminous’ matter we can observe. Studies showed that these two components are related and galaxies (or in general luminous matter) reside inside the dark matter haloes. Thus, in theory by tracing the evolution of the galaxy structures it should be possible to trace the evolution of the underlying dark matter structure. There is however one problem. This dark-luminous matter relation is never straightforward and depends, among others, on the different physical properties of galaxies. In result understanding the relationship between galaxies and the underlying dark matter become one of the key elements and the biggest questions of modern cosmology. The aim and purpose of our project is concentrated around this issue.
At first we address the problem measuring the level of galaxy concentration (clustering). Although this kind of studies have been conducted (extensively) on local Universe data, they have not been performed at early cosmic epoch, when the universe was very young. So seem natural and necessary to extend the analyses.
In this research project we propose an unprecedented study of the large scale structure performed at very early epochs of the evolution of the universe (when it was only 1.5 billions years old). Studies which were previously impossible due to the lack of good enough methods and technology allowing observations of the very weak objects. Now is possible thanks to the first of its type large spectroscopic galaxy survey aimed at observations of the young universe - the VIMOS Ultra Deep Survey (VUDS).
These analyses will allow us to answer the following questions: How, the highly concentrated large scale structure of the universe observed nowadays, has formed and evolved through time? What is the difference between the underlying dark matter density distribution and the observed luminous structures at different cosmic times? What is the physical relation between these two components: dark and luminous, and how this relation changed through time? What processes drive, regulate and truncate the formation of stars inside galaxies? When and what kind of mechanisms dominated the evolution of different galaxy types with given properties at different cosmic epochs. By answering these questions, we want (i) to test if our observations and measurements fit into the modern commonly accepted cosmological model framework and (ii) contribute to the developement of models of galaxy formation and star formation in the early cosmic epochs, which at present are still highly uncertain and lack sufficient observational evidence. This project aims at providing such an evidence.
The level of galaxy concentration can be quantify by measurements of two point correlation function, which will be the very base of all our the studies. This function is based on the very simple idea of measuring the probability of finding two galaxies separated by a given distance. Although the idea is relatively simple, this definition cannot be straightforwardly applied to compute the correlation function from real data samples, because of the limitations of galaxy surveys themselves. In principle, to retrieve ideal correlation functions one would need an access to an unlimited survey which covers the whole sky, and includes all galaxies. Naturally, in practice such surveys are unreachable. So the very first task in our kind of studies is always finding the appropriate method of correlation function estimation. All necessary tools and computer codes have been already prepared and tested by the PI of this project and they are ready for planned new measurements.
Naturally measurement of the correlation function alone would not help us at all. We need to use its approximation in for of accurate and physically justified model. Obtaining the best-fit model parameters allows to interpret the measurements and drive conclusions. There are many prescriptions for such of models. In the first place we are going to use recently the most extensively used ones - so called the halo occupation distribution (HOD) models. However we are also going to challenge the standard construction of the existing HOD models, by modifying their components and possibly adding new parameters. This become a crucial task as the 'standard' prescriptions are slowly ageing. The existing models served (still serve) great in the past decade or so, but they need to be update as there is increasing number of upcoming large galaxy surveys, which statistic will allow to measure very detailed correlation functions. Hence also detailed approximation, without simplified components, is now required.
In conclusion most of planned in this project measurements are going to be among the first ones ever performed with a high accuracy for data form early epochs of galaxy formation. As such, they will provide a valuable benchmark for the interpretation of the co-evolution of galaxies and Large Scale Structure at early epochs of galaxy formation (from the times when the Universe was only 1.5 billion years old) and put constrains on the efficiency of the processes which drive the star formation and mass assembly in galaxies at that time. All of this information can be used, among others, as an input to improve galaxy formation models and simulations, which are still uncertain and need to be informed by improved observational constraints. The measurements and results provided by this project can also be considered as a precursor of next generation studies coming with the advent of the new powerful facilities like e.g. the James Webb Space Telescope (JWST) or Extremely Large Telescopes (ELTs)
Our project will put some new perspective and will improve understanding of the Universe as it was at the early stages of its evolution. For sure our studies will serve as the basis for new follow-up studies, taking us even closer to definitive answers for the unanswered, major cosmological questions.
PRELUDIUM 2016/23/N/ST9/02963 (funded by National Science Centre, Poland)
PI: mgr inż. Małgorzata Siudek
The most common classification of galaxies, proposed by Edwin Hubble in 1926, distinguishes four classes: elliptical and lenticular known as early-type, spiral and irregular, known as late-type galaxies. This classification was only slightly modified later. However, elliptical and spiral galaxies are still considered as two main classes. Elliptical galaxies are usually larger, more luminous, redder and populated by older stars. They are known as “red and dead”, as there is no trace of formation of new stars in them. Spiral galaxies are in contrast smaller, less luminous, bluer and populated by young stars due to still on-going star formation. Thus, evolutionary scenario picturing young, spiral galaxies evolving into mature elliptical galaxies, seems quite natural. However, there is a crucial problem: such an evolution would need more time than the age of our Universe. Moreover, spiral galaxies contain not only young stars but also very old ones. Thus, understanding the evolution of galaxies, and the history of star formation in them, is one of the most important problems in modern astronomy
Astronomers are still searching for a detailed and robust description of the process of galaxy formation and evolution - processes leading to the formation of spiral and elliptical galaxies along radically different evolutionary paths. The fact that complex physical mechanisms regulate these processes is certainly one key contributor to the current state of uncertainty. Another contribution to our lack of understanding can be ascribed to the incomplete census that astronomers have been able to assemble for the properties of galaxies, and for their large-scale distribution, over a large fraction of the 13.5 billion years long history of the Universe. Nowadays we know quite well local Universe – the Universe in the radius of only 2-3 billions of light years from now. The lack of precise measurements of distant, faint galaxies makes it difficult to verify theoretical evolutionary paths of galaxies.
The recently completed VIMOS Public Extragalactic Redshift Survey (VIPERS, http://vipers.inaf.it ), a Large European South Observatory Programme, may change this situation. VIPERS observed almost 100,000 galaxies at 0.5 < z < 1.2, which means that the light traveled from them to Earth for 5-9 billions years. This means that we see these objects as they were at an epoch when the Universe was at approximately half its current age. VIPERS increased significantly the completeness of the census of galaxy properties at those early epochs of the Universe past history providing a unique opportunity to study galaxies and their evolution.
The goal of our project is to study the evolution of red passive galaxies and the history of their star formation over the last 8 billions years. Results of this work will be unique, as so far there was no spectroscopic survey so wide in an area (24 deg2) and reaching beyond z = 1, which means that for the first time the analysis in this redshift range will be so well-grounded from the statistical point of view, and the findings of this project will play a significant role in understanding the evolution of stellar populations in red passive galaxies.
The proposed project is dedicated for studies of a particular type of galaxies: red and passive. This population of massive, elliptical galaxies contains the oldest stellar populations observed in the local Universe. However, astronomers still do not know when and how they formed stars and became quiescent. If we look at the Universe at the half of its age are we also going to observe red and dead galaxies or they will be just in the stage of the formation? Did their stellar populations form during a violent starbust at high redshift or vice versa, they were gradually assembling their mass till relatively low redshift? The project focuses on addressing these questions by spectral and photometric analysis of red passive galaxies observed by VIPERS.
To determine properties and history of star formation in red passive galaxies, I will analyze spectral absorption lines (age and metallicity indicators) and the whole spectra (not only particular lines but also continuum). I will perform also an analysis of broad band magnitudes (from UV to infrared), which allows to describe simultaneously stellar content and dust part (spectral energy distribution fitting). The proposed project is focused on tracing the build up of stellar content in galaxies observed over the last 8 billion years using spectral and photometric information from VIPERS and local surveys (such as SDSS). Finally, I may be able to constrain evolutionary paths leading to formation of red, elliptical and blue, spiral galaxies observed in the local Universe.
HARMONIA 2018/30/M/ST9/00757 (funded by National Science Centre, Poland)
PI: prof. dr hab. Agnieszka Pollo
How galaxies were formed? A simple question, but no simple answer. Even if modern galaxy surveys push our understanding of galaxy physics and evolution further and further, we have no final answer. In the present-day Universe large galaxies are often divided into two basic types. Elliptical galaxies look on the pictures like roundish clouds with no interesting substructure; usually they are filled with old stars and contain no material to form new stellar populations. The other type – spiral galaxies – are those which we most often admire in the pictures made e.g. by the Hubble Space Telescope. They have very complex structure, with spiral arms, bulges, lanes of dust and clouds of gas, dotted by areas where new stars are still being born. These two types are not all yet – the real zoo of galaxies is much more rich. There is a large and mysterious population of lenticular galaxies – “red and dead”, with no new stars forming any more but disk-shaped, often with central bulge, however, with no spiral arms. We have a variety of irregular galaxies, dwarf galaxies and many others. What led to the formation of so different types of galaxies? Some theories say that fate of galaxies was determined when it was born by the properties of the dark matter halo in which it was formed. Other approach attributes a crucial role to interactions with other galaxies. Astronomers agree now that the reality is most like more complex and both these factors played a role in formation and evolution of galaxies. Different combinations of different processes ultimately led to the formation of today’s variety of galaxies.In our project we plan to make use of the unique 3D map of the Universe as it was when it was a half its present age, 8 bln years ago, which we created in the framework of the project VIPERS – VIMOS Public Extragalactic Redshift Survey, which was a Large program of the European Southern Observatory.This map provides us not only the positions of galaxies in the space but also a possibility to investigate their physical properties – masses, shapes, stellar populations - by different means, even if it is obviously much more difficult than in the case of galaxies from our cosmic neighborhood. In our project we will weight and measure these galaxies, compare their properties, classify them, look how they are located in the cosmic web – a sponge-like structure woven of galaxies and dark matter. And we will compare them with galaxies we find in the local Universe, as well as with more distant objects. This will allow us to draw evolutionary paths of different types of today’s galaxies and to understand processes behind them.Shall we catch spiral galaxies turning elliptical – red handed (or at least red)? Shall we see lenticular galaxies just turning red? Huge and unprecedented data of the VIPERS project gives us real chances for such discoveries!
PRELUDIUM 2018/31/N/ST9/03975 (funded by National Science Centre, Poland)
PI: mgr Szymon Nakoneczny
The universe is expanding and the pace of this expansion has been increasing for last several billions of years. The cosmic matter-energy budget is dominated by two mysterious components: dark matter and dark energy. The first one is responsible for the emergence of the large-scale structure of the Universe - the cosmic web of galaxies, galaxy clusters and superclusters, separated by enormous voids. The second one leads to the accelerated expansion - without it, the universe would slow down its expansion due to the attractive nature of gravity.
Those few sentences summarize our current knowledge about the Universe on the largest scales, which we have been gathering for many decades by various observations. This has led to the so-called standard cosmological model, in which few parameters are sufficient to describe the past and the future of our Universe. It is based on known physical laws, especially the most accurate theory of gravity so far - the general theory of relativity. While elegant mathematically and consistent with various astronomical measurements, unfortunately it does not provide an explanation about the nature of dark components, especially the dark energy which is responsible for the accelerated expansion of the Universe.
The goal of this project is to get closer to resolving the dark energy problem, by using two independent, however closely related, types of astronomical observations. The first one is galaxy distribution on the largest scales, about which we learn from large cosmological surveys. The second one is the remnant of the very early and hot stage of the Universe: cosmic microwave background, which was emitted when stars and galaxies did not even exist yet, and the cosmos was filled with a mixture of hot gas and light particles (photons). Studying these two probes, we are able to precisely measure most of cosmological parameters, especially the amounts of dark matter and dark energy, which play key roles in the evolution of the Universe
Large-scale structure and cosmic microwave background characteristics, which we learn about using quite different observational techniques, are inseparably bond together. Photons of the background radiation, travelling through the Universe since the very moment of their emission about 14 billions of years ago, interact in many ways with matter of the large-scale structure. One of such interaction is a change in photons’ energy when they approach large masses such as superclusters of galaxies, as well as their “opposites” - cosmic voids. The same structures also slightly curve photon paths, as a result of relativistic gravitational lensing. The scales of those effects depend on the global amount of dark matter and the rate of cosmic expansion, thus on the dark energy itself. In particular, the net change of background photons’ energy on their way since the emission to us - known as the Sachs-Wolfe effect - would not take place if not for dark energy.
In the proposed project, both the Sachs-Wolfe effect and background radiation gravitational lensing will be used to study dark energy properties as a function of cosmic time. The basic method consists of direct comparison of background radiation and galaxy distribution statistical properties, as well as in studying the imprint of the latter in the former, created as a result of the above mentioned interactions. Precise all-sky background radiation measurements from the Planck satellite will be used together with the largest available galaxy catalogs, which currently include hundreds of millions of objects. Reliability of the results will be ensured by carefully preparing maps and catalogs. The measurements will be eventually compared with many theoretical models which predict different properties of dark energy, as well with those that postulate deviations from general relativity on the largest scales.